Below are a list of OIBL projects:
Motor vehicle collisions are the leading cause of fetal and maternal trauma in the United States. Seatbelts have been primarily evaluated with male Anthropometric Test Devices (ATDs), however, there are large differences in female and male body shape and size. Pregnant anatomy introduces a new set of poorly understood challenges, and there is a significant lack of seatbelt performance testing for pregnant occupants. This research study aims to analyze seatbelt fit with respect to internal and external anatomy of pregnant occupants.
This study involves two phases:
- Internal imaging with an upright Open MRI: Phase 1 collected MRI scans for 6 volunteers at 3 gestational ages seated in a simulated vehicle seat, allowing for seatbelt visualization with respect to internal skeletal and soft tissue structures.
- External 3D imaging: Phase 2 involves imaging 500 participants in 2 environments: the participants are 3D scanned while seated in a simulated vehicle seat, then filmed while seated in their own vehicle; a 3D image is created from this video using photogrammetry.
This study will improve our understanding of seatbelt fit for pregnant occupants, by quantifying anthropometry, belt fit and uterine geometry. Together, the detailed MRI scans and the high volume of 3D images will provide novel information that can advance future developments in injury prediction models and ATDs.
Preliminary Tests for Phase 2- External 3D Imaging
Approximately ~13,000 SCI occur in US and Canada per year and approximately 10% of these injuries occur when the injured person was wearing a helmet. The Incidence rate of Catastrophic cervical injury at high school level 2.56 and 0.68 per 100,000 for Hockey and Football respectively. The overall goal of this project is to design a helmet to prevent spinal and spinal cord injuries (SCI) during head-first impact. Head-first impact involves an inverted fall onto the head (as can happen in hockey, cycling or equestrian sports) or a head-first impact with an object (the roof of a car in an automotive rollover, another player in a football game). These impacts result in devastating consequences such as fracture to the bones in the neck (cervical spine) and contusion or other injury to the spinal cord.
When the spinal cord is injured, paralysis results below the level of injury with devastating physical and financial consequences. In head-first spine impact, the cervical spine will continue to be compressed by the following torso as the torso is decelerated. Depending upon the alignment of the spine, the degree of constraint on the head, and the incoming impact velocity one of three possible outcomes will occur:
- The head will move and allow the spine to flex or extend and escape the incoming momentum of the torso.
- The head will not move out of the way and the spine will resist the momentum of the torso without injury (at velocities less than 3 m/s).
- The head will not move out of the way and the spine will experience injury.
Recent theoretical studies have indicated that some spinal cord injuries can be prevented by guiding the head into a flexion posture during head first impact, which effectively lets the cervical spine "escape" from the path of the large axial load. We have invented and begun to test a two-shell helmet (dubbed Pro-Neck-Tor) that has the capacity to prevent spine and spinal cord injuries during head-first impact by guiding the inner shell and head into flexion or extension with respect to the outer shell. Please see www.pronecktor.com for more information on this project.

Figure 1
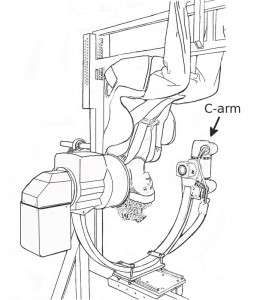
Roll-over Simulation under X-ray
Axial compressive neck injuries occurring during head-first impacts in sports and transportation accidents devastate the lives of those affected and their families. The Injury Biomechanics Laboratory desired a physical surrogate head and neck to provide a sufficiently biofidelic and repeatable response to head-first impacts for the purposes of evaluating injury prevention strategies and testing the efficacy of devices intended to prevent or mitigate injury. A surrogate neck based upon biomechanical concepts designed specifically for head-first impacts has been built for use with a surrogate head and custom drop tower already developed and being used in our lab (Figure 1).
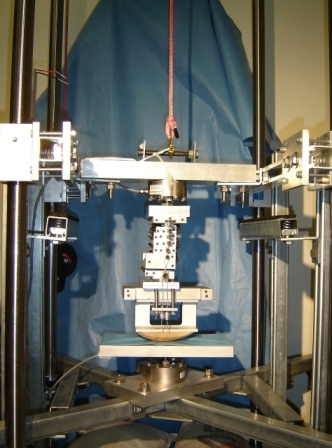
Surrogate head and neck in the drop tower
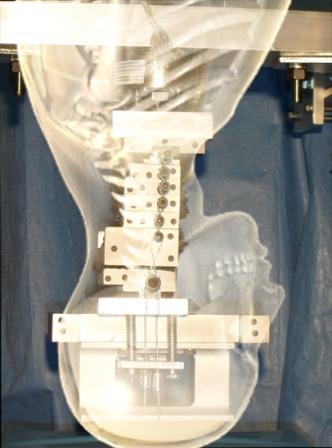
Surrogate head and neck with a superimposed skeletal frame for orientation
The neck design incorporates 8 vertebrae from T1 to C1 in addition to the most superior ‘vertebra’ that represents the occiput or base of the skull. The surrogate head was developed by Phil Morley (see past lab members) and matches the mass and inertial properties of a 50th percentile male human head. The design provides a physiologic range of motion in both flexion and extension rotation, and pure compression allowing motion only in the sagittal plane. Compression is allowed between adjacent vertebrae via slots through which bolts protrude locating the centers of rotation. The intervertebral discs are made of perforated rubber sheets stacked in an offset manner. This provides a non-linear, low initial stiffness that rapidly increases with further deflection for both pure compression and flexion-extension rotation. Each vertebra is constrained to rotate about a center of rotation typically located on an adjacent inferior vertebra. The rubber discs compressing provides resistance to both pure compression and flexion-extension rotation. A spring loaded preload mechanism utilizing 4 cables, 2 on each lateral side, applies follower load along the centers of rotation at each vertebral level. This follower load is intended to simulate the compressive effect of passive musculature in the human neck.
Initial evaluation testing has been performed. The surrogate head and neck are providing repeatable head-first impacts with good biofidelity. The overall and intervertebral ranges of motion have been shown to be in good agreement with in vivo values. However, the measured flexion-extension bending stiffnesses without a follower load are currently more representative of those measured in vitro with a follower load. The initial tests have provided ideas for revisions to improve the biofidelity.
Spinal cord injury occurs at a rate of some 15-40 cases per million population per annum(a), it predominantly affects males in a ratio of 4:1(b) and approximately 50% of people inflicted are under the age of 25(c). The economic, social and personal costs of spinal cord injury are significant to the state, individuals and their families.
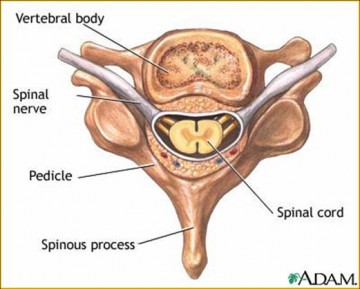
Figure 1: Diagram of the spinal cord and nerve roots with surrounding vertebral body and neural arch (from http://www.adam.com/)
There are several ways in which the spinal cord (see Figure 1) can be injured, including chronic disturbances such as impingement due to tumour growth or disc herniation, or acute injuries such as bone fracture/dislocation and spinal cord laceration, due to sporting accidents or violence.
Understanding the mechanical behaviour of the spinal cord and its associated anatomy during the injury event is important for several reasons. The study of physiological mechanisms of spinal cord injury and potential pharmaceutical agents for treatment require accurate replication of the primary mechanical mechanisms spinal cord injury in animal models. Understanding the mechanics of spinal cord injury and the interactions between different anatomical components will improve the preventative methods and device design, inform physician’s evaluation techniques and treatment decisions, and improve finite element and in vitro modeling.
In collaboration with Dr. Richard Hall (University of Leeds, UK) IBL researchers have recently investigated the effect of cerebrospinal fluid on spinal cord deformation in an animal model of a burst fracture event. The apparatus, consisting of high speed video, a pneumatic projectile system, cryo-clamps and a materials testing machine is shown in Figure 2.
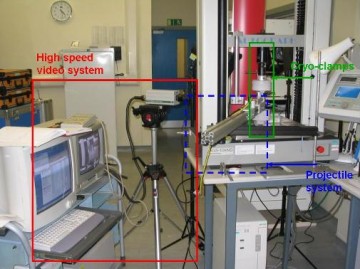
Figure 2: Apparatus for simulating a burst fracture event on a spinal cord specimen. High speed video, pneumatic projectile system, cryo-clamps and materials testing machine. Photo courtesy of Richard Hall, University of Leeds.
The laboratory is continuing the investigation of the role that CSF plays during human injury and how this relates to the injury models currently used to assess clinical interventions. We are specifically interested in the spatial and temporal CSF pressure variation during the injury event.
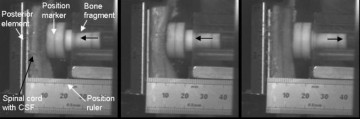
Figure 3: High speed video images of the impact between the simulated bone fragment and bovine spinal cord.
We have also made significant progress in developing a radio-opaque surrogate spinal cord with biofidelic mechanical properties in collaboration with Dr. Richard Hall and Dr. Lynne Bilston (Prince of Wales Medical Research Institute, Sydney, Australia). The cord is shown alongside bovine spinal cord in Figure 4. This has also been used to study the effect of CSF on the biomechanics of the spinal cord injury event. In our laboratory we have also placed Surrogate Cords in cadaver models to measure spinal cord deformation throughout injury event, eliminating the difficulties associated with utilising cadaveric or animal spinal cord. Future work will further improve biofidelity and develop integrated instrumentation.
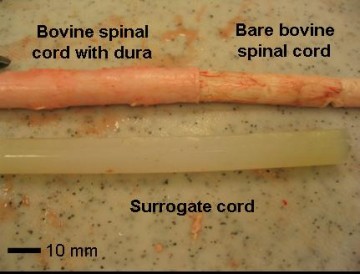
Figure 4: Bovine and surrogate spinal cord specimens.
Current research in spinal cord biomechanics at IBL includes developing methods to measure the deformation characteristics, mechanical properties and interactions of the spinal cord and paraspinal tissues both in vivo, using medical imaging techniques, and in vitro with improved methods of maintaining tissue integrity and biofidelic conditions. This work will have a particular focus on strain rates and load conditions relevant to the injury event.
Funding for these projects has been provided by:
- Canadian Institutes of Health Research, Operating Grant 84375, “The role of cerebrospinal fluid and the subarachnoid space in acute spinal cord injury”
- Commonwealth Scholarship Commission
- British Columbia Neurotrauma Fund
- Rick Hansen Man in Motion Foundation
- International Collaboration On Repair Discoveries (ICORD)
REFERENCES
- Sekhon, L.H.S. and Fehlings, M.G. (2001) Spine 26(24S):2-12.
- Jackson, A.B. et al. (2004) Arch Phys Med Rehabil, 85, pp. 1740-1747.
- Tyroch, A.H., et al. (1997). Archives of Surgery, 132 (7), pp. 778-781.
Research in the area of spinal cord injuries has traditionally been carried out through biomechanical testing where animal spinal cords are exposed and subjected to mechanical injury. We are working on alternative approaches based on medical imaging technologies where MRI and computational image analysis techniques are used to non-invasively assess and study the spinal cord in vivo without exposing the cord. This allows us to study spinal cord deformation and injury in its original physiological environment. This work is done in collaboration with the Biomedical Signal and Image Computing Laboratory (BiSICL) at the Department of Electrical and Computer Engineering, UBC.
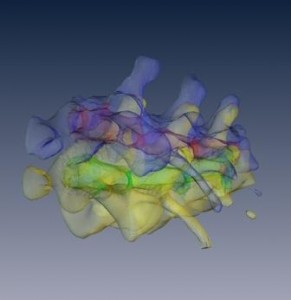
Figure 1: Reconstructed MRI scans of rat thoracic spine segments. Images are presented before and after deformation of the spine.
Fracture of the posterior aspects of the spine, known as spondylolysis (figure 1a), occurs in 6% of the population, and leads to spondylolisthesis (anterior slippage of the superior vertebrae relative to the inferior vertebrae, figure 1b) in 74% of cases. Serious neurological deficiencies can arise due to narrowing of the spinal canal and foramina. Both of these pathologies generally occur in the lower lumbar regions where lumbar motion and shear stresses are highest, and are hypothesized to be related to fatigue loading and deficiencies in the disc, facets and pars interarticularis. The amount of initial slippage at the onset of spondylolysis is also thought to be related to the severity of spondylolisthesis. To date, no functioning model simulating both -lysis and -listhesis exists. The objective of this study is to mechanically create -lysis, followed by -listhesis, on a porcine spine using a physiologic spectrum loading protocol consisting of fatigue and impulse loading.
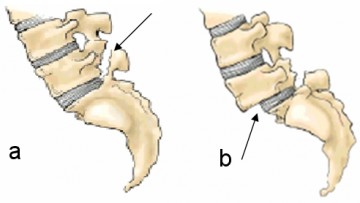
Figure1: Spondylolysis (a) is defined as fracture of the pars interarticularis and leads to Sspondylolisthesis (b) in 74% of cases
In order to produce these pathologies, fresh-frozen porcine functional spinal units (L6/S1 and L4/L5) are being mechanically tested in combined pure shear and compression using uni-axial testing devices. The mechanical setup (figure 2) was designed to mimic anterior displacement of the superior vertebrae relative to a fixed inferior vertebra, with unconstrained motion in the sagittal plane. The loading protocol consists of cyclic fatigue loading interspersed with higher level impulse loads.
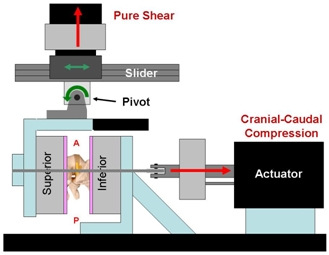
Figure 2: Our testing apparatus is designed to apply a constant cranial-caudal compressive load and a cyclic shear load in order to produce Spondylolysis followed by Spondylolisthesis.
INTRODUCTION
Catastrophic neck injuries are associated with head-first impact in sports accidents and automotive rollovers. These incidents often cause both spinal column and spinal cord injuries. The relationship between the column and cord injury is poorly understood. Clinical information on column and cord injury is valuable but transient conditions such as the velocity of bone fragments, or the transient extent of spinal cord compression can not be elucidated this way. This information could be used to develop novel injury prevention devices or improved animal models.
Additionally, there is a need for an improved axial impact model which accounts for neck musculature. The application of a follower preload has been used in whiplash experiments [1,2] and we hypothesize that it may prevent spine buckling [5] during axial impact.
OBJECTIVE
- Quantify spinal cord deformation continuously during axial impact to the cervical spine.
METHODS
- Human ligamentous cervical spines (Oc-T1) N=6
- A 150N follower preload is applied through two bilateral cables guided along the curve of the spine [3] (Figure 1)
- High speed x-ray (1000fps) imaged a radiodense biofidelic surrogate spinal cord in the canal to provide a sagittal profile
- Custom built drop tower was used to induce axial injury (Figure 2)
- Drop height of approximately 0.5m to obtain the cervical spine tolerance velocity of 3m/s [4]
Two high speed digital video cameras recorded the event (1000 Hz)
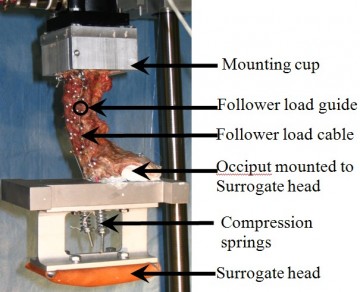
Figure 1: Typical specimen prepared for testing showing follower preload and surrogate head
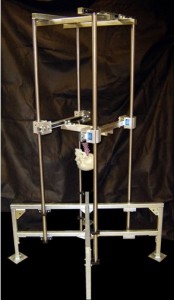
Figure 2: Drop tower used to induce axial impact injuries
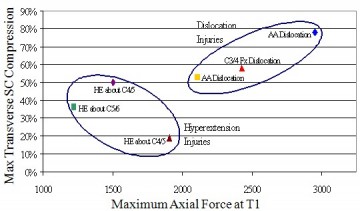
Figure 3: Plot of relationship between transverse SC compression and the axial reaction force
via injury mechanism. (AA – Atlantoaxial, HE – Hyperextension)
DISCUSSION
To our knowledge this is the first time that continuous transient spinal cord deformation could be measured during an axial impact test. A surrogate cord with biofidelic material properties was used. This allowed measurement of cord deformations up to 80%. Simulation of a wider variety of clinical injury patterns are needed to draw statistically significant conclusions about the cord-column relationships. This is the first use of the follower load system to simulate musculature in an axial impact test. Transient buckling was not observed and clinically relevant injuries were obtained.
CONCLUSIONS
- This novel method of quantifying spinal cord deformation allows continuous observation of the spinal cord deformation and can register canal occlusions in excess of 80%.
- Accounting for musculature in an axial impact model appeared to eliminate snap through and complex buckling patterns previously reported [5].
REFERENCES
- Panjabi MM et al. Spine 26:2214-16, 2001
- Ivancic PC et al. Eur Spine J 14: 346-55, 2005
- Hattori SH et al. Z Orthop 119: 568-9, 1981.
- McElhaney JH et al. SAE 790137, 1979
- Nightingale RW et al. JBJSurgAm 78-A: 412-421, 1996.
ACKNOWLEDGEMENTS
Financial support from the Natural Science and Engineering Council of Canada is gratefully acknowledged.
Recent studies have reported an increase in the number of elderly people who are sustaining spinal cord injury (SCI). In the elderly, these injuries often occur in the cervical spine, they often occur in the setting of degenerative spine disease and they often occur in the absence of identifiable fracture or ligament damage. The biomechanics of SCI in the presence of degenerative spine disease in the elderly have not been studied and, as a result, the motions or degenerative features that increase the risk of SCI in this group are unknown. The objective of this study is to examine the effect of spinal degeneration on the risk of SCI in the elderly cervical spine. Experiments will be conducted on cadaveric porcine and human cervical spine specimens (C2-T1). Degenerative features will be simulated on porcine specimens using silicone blocks inserted in the spinal canal. The human specimens will be obtained from elderly donors with advanced spinal degeneration. Physiologic and traumatic bending moments will be applied to the specimens with simulated muscle forces and head weight. Flourscopy will be used to document the deformation of a radio-opaque surrogate spinal cord in the place of the cadaveric cord. An optoelectronic motion analysis system will measure the vertebral kinematics. The relationship between spinal cord compression, vertebral kinematics and degenerative feature size will be measured. From this information it may be possible to develop clinical screening techniques to identify people with high-risk levels of spinal or disc degeneration. The ability to develop prevention or protective strategies such as clincal or environmental interventions that could be used to protect at-risk people (e.g prophylactic stabilization, targeted physiotherapy) may also result from this research.
This project is in collaboration with the Synaptic Analysis Consulting Group, the Department of Orthopaedics (Dina Popovic, M.D., Eyal Itshayak, M.D., Marcel Dvorak, M.D.) at the University of British Columbia, and M.E. Müller Institute at the University of Bern. The Injury Biomechanics Laboratory also acknowledges financial support for this work from the AO Research Grant, the Natural Sciences and Engineering Research Council of Canada, Industrial Postgraduate Scholarship and Synaptic Analysis Consulting Group.
Investigators: F.S. Haddad, M.N. DeHaan, O. Brady, B.A. Masri, D.S. Garbuz, D.J. Goertzen, T.R. Oxland, C.P. Duncan
Femoral fracture in the region of a well fixed hip implant is a difficult problem facing the orthopaedic surgeon. The presence of the implant prevents the use of conventional techniques such as plates and bicortical screws or intramedullary rod fixation. Cortical onlay allograft struts have been increasingly employed in periprosthetic femoral fractures involving a well-fixed femoral stem. With this technique, struts of cortical bone from a donor are fixed on to the exterior of the fractured femur to provide stability (Figure 1). However, there is no standard of practice regarding which fixation variables, such as cable number or strut length, should be selected for optimal fixation results. This study was designed to determine the effect of strut length, strut number and configuration, cable number, cable tension and the effect of cables versus wires on three-dimensional motion at the site of a periprosthetic femoral fracture.
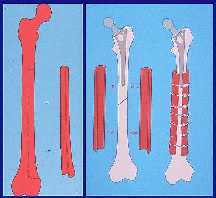
Figure 1
A transverse osteotomy was used to simulate a fracture at the level of the distal tip of a prosthesis. Ten femur-strut constructs were tested in the Instron test machine using a custom designed loading jig. Cranial-caudal and anterior-posterior loads were applied to the head of the femur approximating physiological loading of the femoral head during normal gait. Six degree of freedom motion at the fracture site was measured with the Optotrak system. Figure 2 shows a sagital plane view of a test specimen from the lateral side.
The data show a strong improvement in fracture stability with the use of two struts rather than a single strut alone. However, no difference was detected for the two strut constructs between the medial and anterior strut in combination with a lateral strut. Multifilament cables provide more fracture stability than wires and increasing cable tension gave greater stability. Improved strut fit may explain the decreased motion seen in axial rotation when shortening struts from 20 to 12 cm.
Acknowledgements: Financial support for this work was provided by the George W. Bagby Research Foundation and the John Charnley and BOA/Wishbone trusts and by the Norman Capener Travelling Fellowship. Supplies and equipment were provided by Howmedica (cables and tensioners) and Zimmer (wires and wire tighteners).
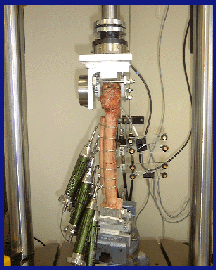
Figure 2
Investigators: A.D. Speirs, T.R. Oxland, B.A. Masri, T.Troczynski, C.P. Duncan The most common cause of hip implant failure is aseptic loosening, which is often the result of osteolytic bone resorption around the implant. Revision of the implant in such a scenario can pose many problems to the surgeon due to the weakness of the femur. A technique introduced by Gie et al.(JBJS, 1993; 75B: p14-21) uses impacted morsellized bone graft to fill the defects, essentially rebuilding the natural structure of the femur. This 'biological' solution has gained popularity because it can restore the patient's bone stock, thus improving the strength of the femur and lowering the risk of another failure. While results have been promising, there is a high rate of complications associated with the technique, including perioperative fracture associated with the trauma of graft impaction and massive subsidence of the implant due to the instability of the graft layer. Furthermore, this procedure is expensive due to the extended operating time and the cost of bone-bank allograft. The long term objective of this research is to develop a suitable alternative to impaction allografting for use in revision hip surgery with massive bone loss. This research is being performed in collaboration with the Departments of Orthopaedics, Mechanical Engineering, Metals and Materials Engineering, Chemistry, Pathology and the Faculties of Dentistry and Pharmaceutical Sciences. Financial support for this work has been obtained from NSERC and the George W. Bagby Research Fund. Aseptic loosening
Revision surgery using the impaction allografting technique. Morsellized cancellous allograft is packed into the femur
Revision surgery using the impaction allografting technique. Morsellized cancellous allograft is packed into the femur to rebuild a "neo-medullary" canal
of a hip implant.
Note the radiolucencies
next to the implant,
a result of osteolytic
bone loss.
Investigators: A.D. Speirs, D. Plausinis, T.R. Oxland, B.A. Masri, C.P. Duncan Trochanteric osteotomy is often performed during a total hip replacement because it offers the surgeon better access to the femur when placing the implant. Since the forces of the hip abductor mechanism are transmitted through the greater trochanter, union of the greater trochanter following the surgery requires adequate fixation. Greater trochanter fixation can be the cause of post-operative complications, depending on the type of fixation, due to cable fretting or breakage or fatigue failure of the wires. This study investigates the in vitro fixation stiffness of different fixation techniques under simulated muscle loads. The osteotomy fragments will be secured to femurs using two different fixation techniques. The loads consist of a varying compressive force perpendicular to the osteotomy plane, simulating the resultant force of the glutei and vastus lateralis muscles, and a shearing force in the anterior or superior direction. Stability will be characterized by the motion of the trochanter relative to the femur, measured with an optoelectronic position sensor. Preliminary results showed that the compressive load affects the amount of motion, and that fixation stiffness varies with direction. Test setup in the Instron.
The GT is fixed to the femur
with the Dall-Miles Cable
Grip System. The rotation
axis of the Instron is used
to apply the compressive load
across the osteotomy site, while
the linear axis pulls anteriorly
on the GT.
Investigators: K.J. Wing, M.F. Dvorak, D.J. Goertzen, T.R. Oxland, C.G.Fisher
Pedicle screws are routinely used in the stabilization of spines affected by tumors, trauma and spondylolisthesis. The pedicle has been shown to account for up to 80% of the pull-out strength of pedicle screws, with the remaining strength coming from the vertebral body. However, like in other parts of the body, conventional screw fixation in osteoporotic bone is poor. We believe that in the setting of osteoporosis, improving the fit and fill of the screw in the pedicle will be a safe and effective method of improving spinal fixation. The objective of this project was to conduct a preliminary evaluation of the fixation characteristics of a novel expandable pedicle screw.
The pilot study compared a standard Synthes USS screw with the new expanding UBC screw (Figures 1 and 2). The USS and UBC screws were inserted in each pedicle of three cadaveric vertebrae. Continuous insertional torque was recorded with an instrumented screw driver. Screws were tested for 100 cycles at three load levels; ±50, 75, and 100N, using the Instron (Figure 3). Three dimensional screw motion was measured using the Optotrak system.
Overall the UBC screw performed well and further testing is underway to compare performance in osteoporotic bone at different vertebral levels. This pilot study established a sound testing methodology and provided an early evaluation of screw fixation. .ICR (Instantaneous Centre of Rotation) for pedicle screws, has not to our knowledge been previously reported. No clear relationship between ICR position and insertional torque or screw toggle displacement was evident. In the future, we will continue testing in the lumbar spine and we hypothesize that in osteoporotic vertebra, the ICR moves dorsally, and that optimizing the fit and fill of the pedicle will improve fixation.
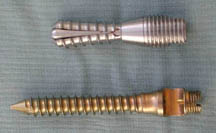
Figure 1
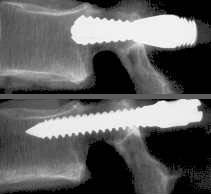
Figure 2
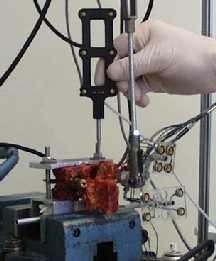
Figure 3
We are looking at the role of neck posture and muscle activation in head first impact (like in a car rollover accident). Our aim is to understand how a person reacts to being upside-down and also how they react before impacting their head. Previous research has shown that the neck alignment and the muscle forces can influence what type of injury a person sustains in this scenario, and certain conditions can put people at a higher risk of spinal cord injury. To help answer this question, we conducted a volunteer study where we secured people in a 5-point harness and turned them upside-down. While they were upside down we measured the muscle activity with EMG and the neck posture with fluoroscopy (dynamic x-rays). By understanding the changes in the mechanics of the neck due to whole body inversion, we hope to improve upon current ex vivo and computational models used to study injury prevention devices.
Neck, or cervical spine, injuries often have devastating consequences for the afflicted individuals with quadriplegia or paraplegia resulting from the most severe injuries. Most neck injuries do not result in paralysis but do present a challenge to the physician regarding the best treatment protocol. The treatment may range from surgery with stabilizing implants, to external stabilization using a halo vest or neck collar, to no intervention. The treatment decision is based upon the judgement of the presiding surgeon regarding the integrity of the spine and the relative likelihood of progressive neurological injury, deformity, or pain. The integrity of the spine is assessed by radiographic techniques such as X-ray, CT, or MRI scanning and the hypothesized injury mechanisms. Unfortunately, the relationships between the injury mechanisms, the tissue damage, and the resultant biomechanical flexibilities have not been established experimentally. The goal of this research work is to carefully study the mechanisms of cervical spine injury at high loading rates and relate the anatomic integrity of the injured spines to their flexibility. The findings of the work will form a basis to support the clinical decision making in the treatment of neck injuries. Preliminary experiments are underway in variety of sub-projects.
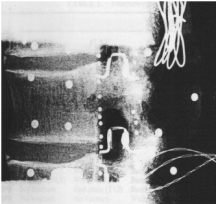
Cervical spine specimen with
implanted canal encroachment
transducer [Panjabi et al. 1995]
We have forged collaborations with the University of Washington in Seattle (Dr. Randy Ching), the University of Saarland in Homburg, Germany (Dr. Tobias Pitzen), Thomas Jefferson University in Philadelphia (Dr. Alex Vaccaro), and the First Military Medical University in China (Dr. Qingan Zhu) that will enhance substantially our efforts in this research program.
Financial support for this work has been obtained from NSERC, the Rick Hansen Institute, and the Canadian Orthopaedic Foundation.
Previous publications on neck injury:
Panjabi MM, Kifune M, Liu W, Arand M, Vasavada A, Oxland TR. Graded thoracolumbar spinal injuries: development of multidirectional instability. Eur Spine J 1998; 7(4):332-9.
Kifune M, Panjabi MM, Liu W, Arand M, Vasavada A, Oxland T. Functional morphology of the spinal cord after endplate, wedge, and burst fractures. J. Spinal Disorders 1997; 10:457-466.
Lin RM, Panjabi MM, Oxland TR. Significant roentgenographic parameters for evaluating the flexibility of acute thoracolumbar burst fractures. Int. Orthop. 1997; 21:109-114.
Panjabi MM, Kifune M, Arand M, Wen L, Oxland TR, Lin RM, Yoon WS, Vasavada A. Dynamic Canal Encroachment During Thoracolumbar Burst Fractures. J. Spinal Disorders 1995; 8:39-48, 1995.
Oxland TR, Panjabi MM. The Onset and Progression of Spinal Injury: A Demonstration of Neutral Zone Sensitivity. J. Biomech. 1992; 25(10):1165-1172.
Oxland TR, Panjabi MM, Southern EP, Duranceau JS. An Anatomic Basis for Spinal Instability: A Porcine Trauma Model. J. Orthop. Res. 1991; 9(3):452-462.
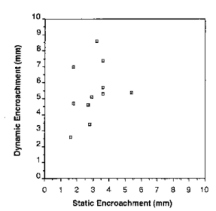
Graph showing canal
encroachment as measured
by the transducer
vs a static CT scan.
Dynamic compression
measurements show that
CT scans underestimate the
amount of impingement of
the spinal cord
[Panjabi et al. 1995].
Investigators: C. Lane, T.R. Oxland, S. Tredwell, B. Sawatzky
Scoliosis is a spinal deformity characterized by an abnormal curvature in the coronal plane and an axial rotation. Scoliosis surgery aims to correct this three-dimensional deformity by inserting rods and hooks to hold the spine while fusing the vertebra in a physiological position. Working with Dr. Steve Tredwell and Dr. Bonnie Sawatzky at Vancouver's Children's Hospital, we are comparing intra-operative three dimensional spinal analysis, radiographic data, spinal shape analysis and pelvic motion analysis to develop strategies that will maximize the spinal correction and minimize the number of vertebral levels that need to be fused.
The intraoperative measurements of spinal deformity will utilize a precision optoelectronic camera system (Optotrak 3020, Northern Digital, Waterloo CAN) and a locally defined anatomical coordinate system. This camera system measures the three-dimensional position of infrared LEDs with an accuracy of less then 0.1mm in a plane parallel to the camera and less than 0.15mm in the direction towards and away from the camera. We are also including functional assessment of all patients before and after surgery such that we can connect the surgical procedure with the outcome. Our rationale for this approach is to specifically focus on the long term goal of limiting the extent of fusion surgery in the lumbar region while maintaining the efficacy.
Financial support for this work has been obtained previously by Dr. Tredwell from the Medical Research Council of Canada.
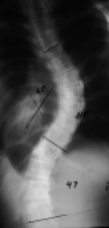
Pre-operative severe right
idiopathic scoliosis, later
treated using a segmental
correction system modeled
from the Cotrel-Dubousset
instrumentation system.
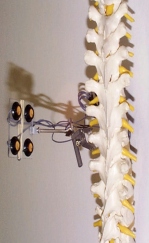
Prototype Optotrak marker
carrier mounted on a spine
model.
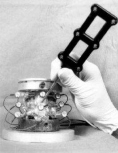
The Optotrak Digitizer that
is used to define the
anatomical reference frame.
Investigators: D.J. Goertzen, T.R. Oxland, J.P McConkey
The effect of complete ligament rupture on joint function has been studied extensively. Much less work has been done on partial or sub-failure injuries, presumably since clinical diagnosis is more subtle and the clinical situation less acute. This type of lesion may be caused by a single, traumatic event or by repetitive low-level strain. Biomechanical studies have documented that overstretched ligaments result in increased joint laxity. The critical injury levels and internal mechanisms remain unknown although studies have suggested values between 4 and 10% strain for the onset of permanent ligament laxity.
We are in the early stages of this project and the areas of interest are in both the mechanical and histological realms and how they relate. The objectives of this work are to study the mechanism of subfailure tendon and ligament injuries, the factors which affect their severity, and the underlying structural changes in the tissue.
Rabbit medial collateral ligaments (MCL) are being used to investigate the mechanical characteristics of the partial injury of the ligament. Figure 1 shows a rabbit femur and tibia mounted in our custom tensile tester with the MCL exposed. Paired tests were carried out where one ligament was loaded to produce a sprain and the other ligament served as a control with no overload applied. Mechanical evidence of the sprain is evident when two initial loading curves are superimposed. The overloaded ligament exhibits a permanent deformation which corresponds clinically as joint laxity (Figure 2). We are investigating a number of factors that affect this type of injury.
We are also looking at the tissue histology and investigating relationships between mechanical evidence of a partial injury and the histological appearance of the same tissue. This information would help in the treatment and prevention of these common injuries.
Financial support for this work was obtained from The B.C. Medical Services Foundation/Vancouver Foundation.
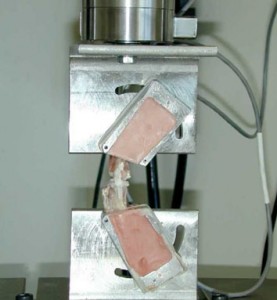
Figure 1
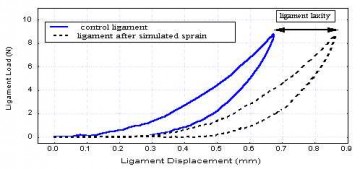
Figure 2
Investigators: A. Choo, T.R. Oxland, W. Regan, D. MacIntyre
Isokinetic testing is a common rehabilitation technique for patients after shoulder surgery. However, current approaches do not ensure that the two primary joints of the shoulder complex (i.e. glenohumeral and scapulothoracic) move in any consistent pattern and precise movement of these joints is largely unknown due to difficulty in tracking the scapula.
Objectives:
- To develop an accurate, non-invasive, repeatable means of measuring glenohumeral and scapulothoracic motion.
- To quantitatively evaluate surgical success, rehabilitation regimes, identify mechanisms of shoulder pathology and provide a means of assessing analytical models of the shoulder joint complex.
Roentgen stereophotogrammetric analysis was selected as the "gold standard" for determining in vivo rigid body kinematics. This involves involves the implantation of 0.8-mm metalic (tantalum) markers into bone. The markers/bones are image with stereo x-ray and their 3-D coordinates are thus determined. A system of surface skin markers will be used to correlate the deformable motion of the skin with the underlying bone.
This project is still in progress but preliminary results indicate that skin markers are a promising means of tracking scapular and humeral motion.